Entanglements Are Good—at Least in This Case
Explaining the mysterious phenomenon of Quantum Entanglement and it's crucial role in Quantum Computing
QUANTUM LEARNING
Surya Ravichandran
6/21/20235 min read
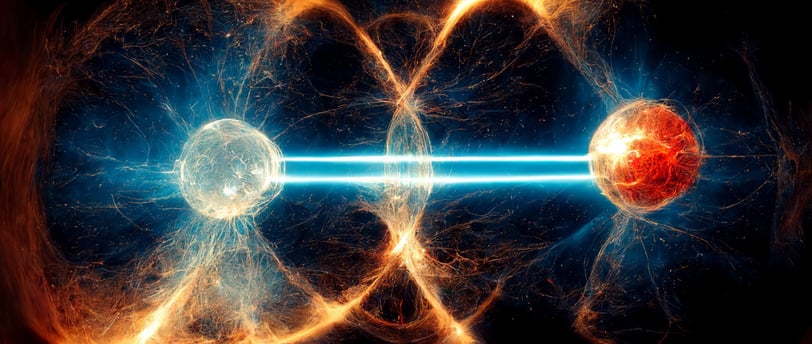
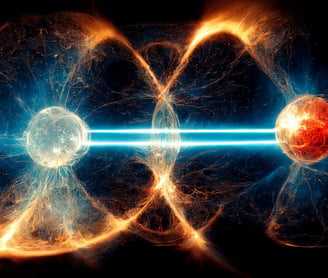
Quantum entanglement is like a mind-bending puzzle within the mind-bending world of quantum mechanics. It's something that has fascinated scientists since it was discovered. This strange phenomenon challenges our usual understanding of reality and has big implications for technology and our understanding of the universe. In this article, we'll dive into how quantum entanglement was discovered, explore its mind-boggling properties, and try to explain it in a way that makes sense to beginners and those unfamiliar with the concept.
The History of Quantum Entanglement
Think back to 1935 when Albert Einstein, Boris Podolsky, and Nathan Rosen came up with something called the EPR paradox. It was a thought experiment where they noticed when particles were entangled, they acted in really weird ways. It was like they were instantly connected, no matter how far apart they were. This clashed with what classical physics predicted. Over time, scientists dug deeper, and now quantum entanglement is a key part of quantum mechanics. It turns out that entangled particles, whether they're atoms or photons or other quantum systems, have a special bond where their properties are linked, no matter the distance between them. Let's take a deeper dive into this crazy phenomenon, and try to make some sense out of it
The spin can be up, down, or any which way around; The relationship between Quantum Computing, Quantum Entanglement, and Electron Spin:
In the world of quantum mechanics, the behavior of particles can be bewildering. One intriguing property is the spin of electrons, which plays a significant role in the fascinating fields of quantum computing and the concept of quantum entanglement.
Electron spin is a unique property that describes how an electron "rotates," but it's not quite like any familiar spinning object we encounter in our everyday lives. Instead, it's a quantum property that represents the electron's internal angular momentum. Depending on how the electron's spin is oriented, it can exist in either a higher or lower energy state. Think of electron spin as a kind of internal twirling motion, but on a minuscule scale that defies our intuition. This spinning-like behavior, or "spin," has a significant impact on the electron's energy level. It's as if the direction in which the electron is "twirling" determines whether it has more or less energy.
In terms of electron spin, there are two fundamental states: spin up and spin down. When an electron is in its spin-up state, it implies that its spin is aligned in one direction, typically designated as +1/2. On the flip side, in the spin-down state, the electron's spin is aligned in the opposite direction, often represented as -1/2. These spin states correspond to specific energy levels, and the relationship between spin and energy plays a crucial role in quantum phenomena.
Quantum entanglement, a phenomenon Einstein famously referred to as "spooky action at a distance," is closely related to the electron spin. When multiple particles, including electrons, become entangled, their quantum states become intrinsically linked. For instance, two electrons with opposite spins can be entangled such that measuring one electron's spin instantly determines the spin state of the other, regardless of their separation. This entanglement manifests as a correlation between the spins of the entangled particles, even though they may be far apart.
Let's consider an example to understand this concept better. Imagine we have two electrons, let's call them Electron A and Electron B. Initially, Electron A is in a spin-up state (+1/2) while Electron B is in a spin-down state (-1/2). When these electrons become entangled, their spins become intrinsically linked, as if they are connected by an invisible thread.
Now, let's separate Electron A and Electron B by a large distance. It could be kilometers or even light-years apart. Surprisingly, the entangled nature of their spins allows for an extraordinary correlation. If we measure the spin of Electron A, instantaneously, we will know the spin state of Electron B, regardless of how far apart they are.
The remarkable connection between electron spin and quantum entanglement has opened up new frontiers in computational power and quantum computing. In quantum computing, qubits, the building blocks of quantum information, can be realized using electron spins. By manipulating the spin states of qubits, complex quantum computations can be performed.
Quantum algorithms leverage the power of entangled electron spins to solve problems exponentially faster than classical algorithms. For instance, Shor's algorithm utilizes quantum entanglement to factor large numbers quickly, which has significant implications for cryptography. Similarly, Grover's algorithm utilizes entanglement to accelerate database search operations. These algorithms harness the interplay between electron spin and entanglement to explore parallel computational paths and achieve remarkable computational efficiency.
Get ready to jump into the mind-blowing story of Peter Shor and his game-changing algorithm! Check out the quick 5-minute video on YouTube (on the left) to get all the juicy details. And if you're feeling up for it, dive into the longer version (on the right) for a more immersive experience. Trust me, you're in for a wild ride with Shor's algorithm, a true showcase of human brilliance.
Quantum Computing in Context
To better understand the power of quantum entanglement and electron spin, consider a quantum computer simulating molecular interactions. Researchers utilize the unique properties of entangled electron spins to achieve this feat.
By representing molecules as collections of qubits, where each qubit corresponds to an electron spin associated with a specific atom or molecular component, quantum computers can simulate complex chemical reactions. The entanglement of electron spins enables the exploration of numerous molecular configurations simultaneously, providing valuable insights into reaction rates, energy levels, and the behavior of electrons within molecules. This quantum approach to simulating molecular interactions holds the promise of revolutionizing fields such as materials science and drug discovery by enabling the study of larger and more complex systems that exceed the computational capabilities of classical computers. While challenges remain, the power of entangled electron spins in quantum computers opens up new frontiers in understanding and manipulating chemical processes at the molecular level.
If you'd like to see an actual study donducted on molecular simulation, here is one to look at and read through.
Wrap up
I know we've been delving into the Physical aspects of Quantum technology so far, but trust me, this knowledge is going to have some real-world applications very soon. In the upcoming posts, we're going to switch gears and explore the practical side of quantum computing and how it can revolutionize technology, business, and our everyday lives.
Now, before you start picturing quantum gadgets on store shelves, let me be honest – we're not quite there yet when it comes to quantum technology becoming a part of our daily lives. But here's the exciting part: there are already companies and research facilities out there using quantum technologies to push the boundaries of what's possible in their industries. They're making big strides behind the scenes.
In our next post, we're going to dive headfirst into the world of qubits. Don't worry if you're not familiar with the term – we'll explain it all. We'll break down how qubits differ from the traditional bits we're used to, and we'll go into detail about how these incredible quantum components are measured. Brace yourself, because some truly mind-blowing stuff is on the way. Keep an eye out for our next update!
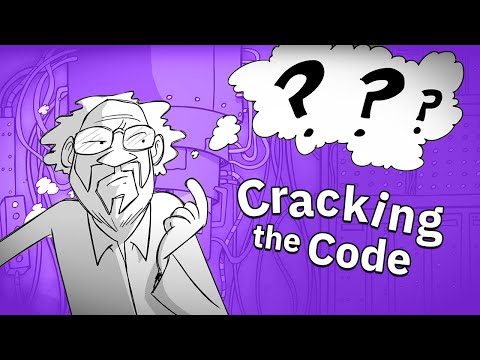
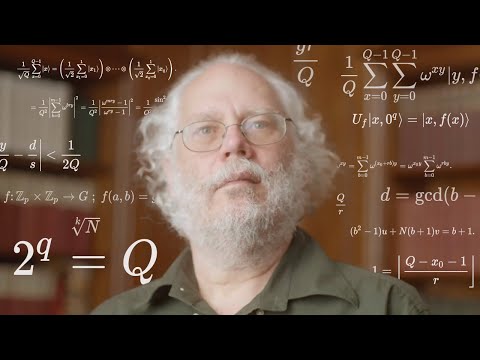
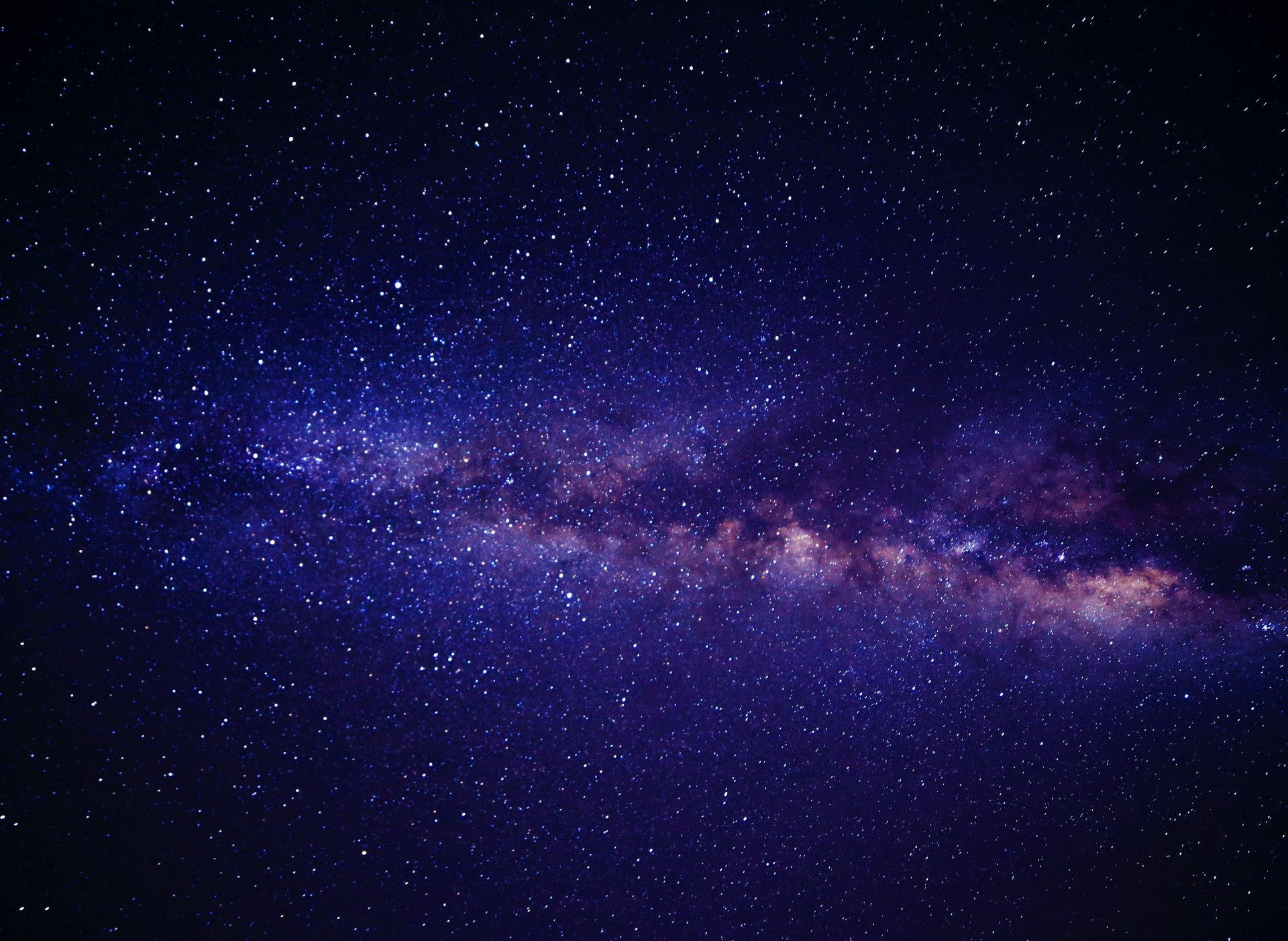